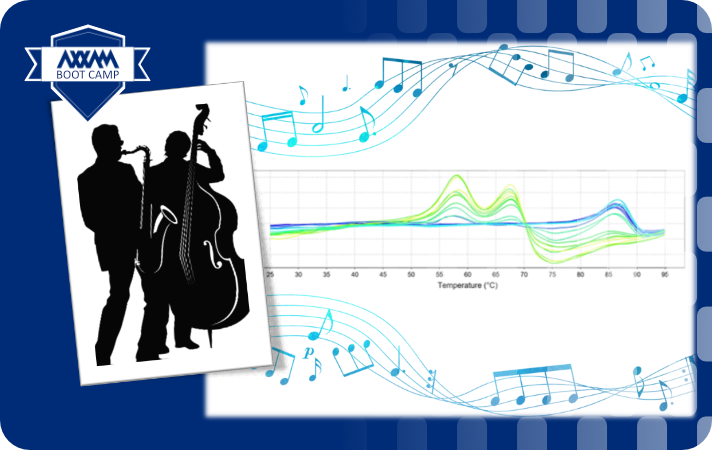
Thermal Shift Assays meet HTS to discover and characterize novel protein binders
Thermal-shift assay (TSA) represents a powerful tool for protein-stability analysis and for discovering and characterizing new protein binders. Understanding protein stability is crucial for drug development, protein engineering, and other biotechnology applications. Among the various methods available for assessing protein stability, TSA has emerged as an effective technique. TSA provides valuable insights into protein stability, ligand interactions, and quality control, making it indispensable for drug development and protein engineering. Here, we outline the principles, methodology, and applications of TSA, as well as our recommendations for use in early-stage drug discovery and our TSA capabilities to identify and characterize protein binders for your target protein.
TSA is a highly parallelizable, cost-effective technique for studying protein stability and protein binders. It measures the thermal denaturation of a target protein by monitoring the fluorescence intensity of a dye that binds to exposed hydrophobic regions of the protein during heating. As the protein unfolds, the exposed hydrophobic regions increase, leading to an increase in fluorescence signal. Compounds, such as inhibitors or in general modulators, stabilize the three-dimensional conformation of the target protein and as a consequence the temperature needed to denature the protein is higher (see purple curve in left figure).
The figure on the right below shows fluorescence intensity curves (top panel). The blue curve is the target protein with the dye; the other traces correspond to the addition of increasing concentration of a binder compound that causes a shift of the melting temperature of the protein complex. The melting temperature can be calculated as the inflection point of the fluorescence intensities or as the first derivative of the curves (peaks in the bottom right panel).
TSA and differential scanning fluorimetry (DSF) can generally be used interchangeably, a primary distinguishing feature between the two lies in their denaturation methods. TSA specifically refers to the denaturation of a target protein triggered solely by temperature intensification. On the other hand, DSF encompasses techniques that employ various physicochemical agents, including but not limited to temperature intensification, in order to induce denaturation in the target protein. In this article, our focus will be directed towards exploring the specific aspects of TSA.
The TSA methodology involves a series of simple steps that make it suitable for routine laboratory use. The following is a brief outline of the key steps involved in performing a TSA experiment:
Overall, TSA offers numerous applications in various fields, including:
Additionally, TSA enables the ranking of compounds based on their affinity to the target protein, providing valuable insights into their binding properties. By using TSA, researchers can confidently evaluate compounds and identify those with a higher or lower affinity to the target protein, aiding in drug discovery and optimization processes.
When considering the use of TSA in early-stage drug discovery, we offer the following recommendations:
One of the notable advantages of TSA is its ability to create a cell-free, label-free, and immobilization-free simplified environment for studying protein-ligand binding and assessing the effects of protein mutations. Because target proteins do not require labelling in TSA, the experimental setup is straightforward. This feature allows for a streamlined and efficient workflow, saving time and resources.
The main limitation of TSA is that it relies on the assumption that protein unfolding is accompanied by an increase in exposed hydrophobic regions, which may not always hold true. Certain protein classes may have complex unfolding profiles that cannot be accurately measured using TSA alone. In such cases, combining TSA with complementary techniques can provide a more comprehensive understanding of protein stability.
Moreover, although TSA is suitable for most target protein classes, it is important to note that it may not be ideal for studying membrane-bound proteins, such as G-protein coupled receptors (GPCRs). GPCRs, for example, have naturally exposed hydrophobic regions which allow the immediate binding of the fluorescent dye used in TSA, thus creating an unusually high fluorescence signal and preventing normal fluorescence curve readouts upon increasing temperature. Alternative techniques and/or fluorogenic dyes should be considered for such protein classes.
While TSA has its limitations, it remains a versatile technique that continues to contribute significantly to the advancement of biopharmaceutical research. By harnessing the power of TSA, new therapeutic targets can be uncovered, and more effective protein-binding drugs can be developed, bringing us closer to improved healthcare outcomes.
At Axxam, we offer a range of TSA capabilities to support your research needs:
By leveraging our TSA capabilities, you can benefit from our in-house protein production, advanced instrument platform, and screening and profiling options. We are committed to providing reliable and efficient TSA services to support your research and drug discovery endeavors.
Thermal Shift Assays meet HTS to discover and characterize novel protein binders